You’ve probably seen them.
Those tiny bugs that hover over your fruit bowl in the summer if your bananas get too ripe.
While your first reaction might be to smush these kitchen invaders, most folks don’t realize some of the most important biological discoveries of the last 150 years were made by scientists studying this organism, including those that have had a direct impact on human health.
A biomedical revolution, led by bugs
Realizing just how much we have in common with the fruit or vinegar fly (Drosophila melanogaster, above), can be surprising. Sure, there are lots of differences on the outside (hey, we don’t have wings), but at the level of molecules, genes and cells, fruit flies and humans share many similarities. For example, a fly’s eye develops in much the same way our own eyes do, using the same set of genetic instructions, cellular machines, and cell types to make a highly complex organ. In fact, the fly eye develops just fine using either its own eye gene or one from a mouse!
This biological similarity is extremely important, because what we learn about how genes, cellular machines, and cells work in fruit flies can tell us how they work in humans, too. Since using humans for certain experiments is unethical, fruit flies are invaluable to biomedical research. People tend to not mind if you sacrifice an alien-looking, slightly annoying bug for the greater good of humankind.
Fruit flies are model organisms because they serve as a proxy for understanding human biology. My molecular biology colleagues use frogs, mice, and worms that have their own experimental advantages.
I use flies because they are easy to grow (an over-ripe banana works in a pinch), have a very fast generation time (egg to adult in 10 days), produce thousands of offspring, and have lots of genetic tools that allow us to figure out just how cellular machines work. Imagine if cattle and sheep had the same biological advantages as flies (fast generation time, simple dietary requirements). Ranch productivity would be sky-high (and more money in a rancher’s pocket)!
Harnessing the power of model organisms to understand human disease
My research leverages these advantages in the fruit fly to understand the molecular basis of human disease. My goal is to determine how mutations in genes disrupt the function of cellular machines and, in turn, alter cell behavior that ultimately leads to disease.
Once we know how this process works at its most basic level, other scientists can use this information to design better therapeutic treatments.
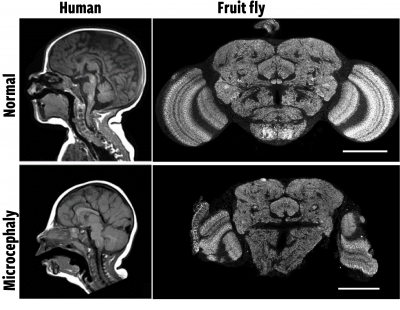
My lab focuses on microcephaly, characterized by a reduction in brain size, intellectual disabilities, and early death (Figure 1). While doctors understand the clinical aspects of the disorder, we don’t know the molecular details: how do genes instruct the brain to develop to the correct size? We know the human gene Abnormal Spindle-Like Microcephaly-Associated Protein(ASPM) is frequently mutated in microcephaly patients, but we don’t know what it does in cells that ultimately leads to small brains when it’s not working.
This was the question I wanted to address as a researcher at the National Institutes of Health. Flies have their own ASPM gene version, so I used gene-editing technology to create a mutation in the fly ASPM gene. This led to fruit flies that had small brains, exactly what we see in human microcephaly patients (Figure 1).
Fruit flies build brains the same way humans do
I then performed experiments to figure out what was going wrong in brain cells that had a mutant ASPM gene. My preferred tool is light microscopy, because we can see exactly what cells and their cellular machines are doing as a fly brain develops (Video 1). I found the cellular machine that helps a cell divide into two cells, known as the mitotic spindle, was defective in a special population of brain cells: the neural stem cells (Video 2).
This was an exciting finding, because that told me that ASPM’s job was to ensure the mitotic spindle was working correctly so cells could multiply. But what was the link between this cellular defect and small brains?
The brain is a complex organ, whose size is thought to be determined by the number of cells present. In humans, there are about 170 billion brain cells! However, these cells must come from somewhere since we all start life as just a single cell. During development, cells divide to increase cell number, and the organism grows as a result. This is the job of the neural stem cells: divide multiple times using the mitotic spindle machinery to make all the brain cells.
Based on this logic, I hypothesized microcephaly was the result of mitotic spindle defects occurring in neural stem cells due to mutations in ASPM, which prevented them from dividing properly. Fewer cells equals smaller brains (Figure 2).
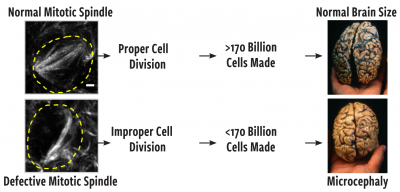
Putting the hypothesis to the test
If the hypothesis was true, then we should see small fly brains whenever we see defective mitotic spindles. To test this, I performed a “genetic rescue” experiment, where I added back different parts of ASPM to mutant flies and looked for both mitotic spindle and brain size defects, the latter of which I measured using microcomputed tomography (µ-CT), the perfect tool for visualizing small fly brains (Video 3).
The result was not what I expected. Amazingly, only a small piece (~1/4) of ASPM was needed to make a properly sized brain – and it could still do this even though the mitotic spindle was defective in the neural stem cells. Apparently, brains don’t care if the cell division machinery is not working quite right; they still find a way to grow to the correct size anyway (Figure 3)! The data simply didn’t support my hypothesis.
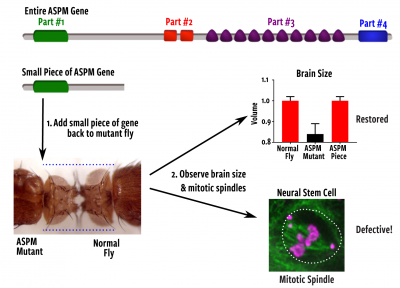
Failure in science as a path to discovery
Failure is a common occurrence in science. We fail to support our hypotheses or get experiments to even work. Rather than being a series of successful experiments that lead you right to the answer, science is more like fumbling around in the dark, hitting dead ends along the way, until you finally emerge on the other side with only a few answers.
But that’s the beauty.
Science might be the only profession where failure actually gets you anywhere. It’s the combined action of many scientists, making small advances in knowledge despite these failures, that build the forest from the trees. And often, the data that failed to support your hypothesis ends up telling you more about what’s going on than you could have ever imagined.
In my case, that’s true. Based on the data from that experiment and a few new ones, my lab is now on the cusp of figuring out exactly what this small piece of the ASPM gene does, the cellular machines involved, and how it controls cell behavior so a brain grows to its correct size.
Who knew a tiny bug who loves rotting fruit could be so powerful?
What the fruit fly can tell us about cancer
The fruit fly also serves as an excellent model for understanding cancer, the second-leading cause of death in the US.
Cachexia is a muscle wasting phenotype seen in many terminal cancer patients, often directly responsible for death. Through my collaboration with Dr. Tor Erik Rusten at Oslo University Hospital in Norway, we recently uncovered how cachexia happens at the molecular level. Cancer cells can trick normal muscle cells into turning on a nutrient recycling program called autophagy, which breaks down muscle protein into amino acids.
These amino acids are then taken up by tumor cells to fuel their growth. By turning off autophagy in fly muscle cells, we could prevent muscle tissue from breaking down and tumors from growing, essentially stopping cancer in its tracks. This information can now be used to design better therapeutic strategies or drugs to treat human cancer.
To contact: Schoborg can be reached at (307) 766-3527 or at todd.schoborg@uwyo.edu.
Related videos
Video 1. Video of a Drosophila brain developing in real time, as observed by light microscopy. The different types of cells in the brain are labeled, including neural stem cells, neurons, and glia. The neural stem cells divide multiple times to make all the neurons and glia of the brain. In this video, a neural stem cell undergoes two rounds of division to illustrate this process. Video credit: Nasser Rusan, National Institutes of Health.
Video 2. The mitotic spindle, the cellular machine that allows neural stem cells to divide and make neurons and glia, is shown in both a normal stem cell and an ASPM mutant stem cell. One cell division is shown. Note how the mitotic spindle in normal cells forms rapidly into a tight diamond shape, followed by rapid separation as it moves the chromosomes into each daughter cell. ASPM mutant mitotic spindles fail to maintain their diamond shape and there is a long delay until the chromosomes finally separate into the daughter cells. Time is shown in minutes.
Video 3. Micro-computed tomography (µ-CT) of an intact fruit fly, rendering in 3D. Most of the fly has been made “transparent” and then digitally cut away to show the central nervous system. The ventral nerve cord, like the vertebrate spine, is shown in yellow. The entire brain, located in the headcase, has been colored to show the sub-regions responsible for vision (optic lobes, blue) and learning and memory (central brain, red). This tool is used to precisely measure brain size in small flies.
Note: These videos and others are available on the Wyoming Agricultural Experiment Station’s YouTube channel at bit.ly/youtube-WAES.